Subatomic Physics
Nuclear physics in supernovae
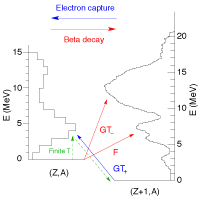
Nuclear physics is a basic ingredient for supernova simulations. When the nuclear fuel for fusion in a star is emptied, its core starts to collapse. The electron gas is compressed and electrons in higher momentum states are captured on free protons and increasingly heavy nuclei. Neutrinos are emitted. This deleptonization becomes limited by rising neutrino opacities as the density increases during collapse. The homologously collapsing inner core bounces when nuclear densities are reached. The energy of the bounce shock is determined by the size of the inner core and the stiffness of the equation of state, which determines the pressure of nuclear matter as a function of temperature, density, and composition. However, the kinetic energy of the shock is quickly dissipated by the emission of neutrinos and dissociation of the still infalling nuclei of the outer core. The shock front comes to a stall and the detailed mechanism of revival to produce a supernova explosion remains unclear. It is a question of the geometry and the interaction between neutrinos and nuclear matter how efficiently heating through the absoprtion of escaping neutrinos supports further shock propagation. This project does not directly develop nuclear physics improvements for the supernova context. In collaboration with Nuclear Physics groups it explores the sensitivity of the models to suggested improvements in various places. Some of our views on the role of nuclear physics in core collapse supernovae are expressed in this Nucl. Phys. A proceedings article or this more detailed Nucl. Phys. A review article.
Supernova yields from proton-rich ejecta
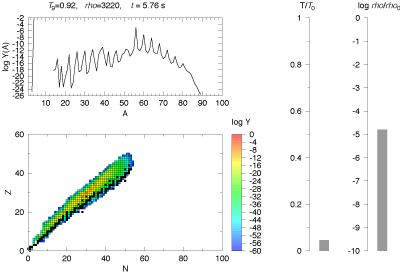
In a long-term collaboration with the group of Prof. Thielemann and external experts the following improvements of nucleosynthesis predictions were obtained(Fröhlich, C., Hauser, P., Liebendörfer, M., Martínez-Pinedo, G., Thielemann, F.-K., Bravo, E., Zinner, N. T., Hix, W. R., Langanke, K., Mezzacappa, A., & Nomoto, K. 2006, ApJ, 637, 415):
"With currently known input physics and computer simulations in one dimension, a self-consistent treatment of core-collapse supernovae does not yet lead to successful explosions, while two-dimensional models show some promise. Thus, there are strong indications that the delayed neutrino mechanism works combined with a multidimensional convection treatment for unstable layers (possibly with the aid of rotation, magnetic fields and/or still existent uncertainties in neutrino opacities). On the other hand, there is a need to provide correct nucleosynthesis abundances for the progressing field of galactic evolution and observations of low-metallicity stars. The innermost ejecta is directly affected by the explosion mechanism, i.e., most strongly, the yields of Fe group nuclei for which an induced piston or thermal bomb treatment will not provide the correct yields because the effect of neutrino interactions is not included. We apply parameterized variations to the neutrino-scattering cross sections in order to mimic in one dimension the possible increase of neutrino luminosities caused by uncertainties in proto-neutron star convection. Alternatively, parameterized variations are applied to the neutrino absorption cross sections on nucleons in the ``gain region'' to mimic the increase in neutrino energy deposition enabled by convective turnover. We find that both measures lead to similar results, causing explosions and a Ye>0.5 in the innermost ejected layers, due to the combined effect of a short weak-interaction timescale and a negligible electron degeneracy, unveiling the proton-neutron mass difference. We include all weak interactions (electron and positron capture, beta-decay, neutrino and antineutrino capture on nuclei, and neutrino and antineutrino capture on nucleons) and present first nucleosynthesis results for these innermost ejected layers to discuss how they improve predictions for Fe group nuclei. The proton-rich environment results in enhanced abundances of 45Sc, 49Ti, and 64Zn as required by chemical evolution studies and observations of low-metallicity stars, as well as appreciable production of nuclei in the mass range up to A=80."
"We present a new nucleosynthesis process that we denote as the νp process, which occurs in supernovae (and possibly gamma-ray bursts) when strong neutrino fluxes create proton-rich ejecta. In this process, antineutrino absorptions in the proton-rich environment produce neutrons that are immediately captured by neutron-deficient nuclei. This allows for the nucleosynthesis of nuclei with mass numbers A>64, making this process a possible candidate to explain the origin of the solar abundances of Mo92,94 and Ru96,98. This process also offers a natural explanation for the large abundance of Sr seen in a hyper-metal-poor star."
These results have been very well received and discussed in several places:
- A new way to make elements Physical Review Focus
- Neutrinos provide new route to heavy elements in supernovae Cern Courier
- Nuclear physics: Extending the elements Research Highlights : Nature
Data needs for nuclear astrophysics
Astrophysical simulations strongly rely on subatomic input physics. The spread of conditions assumed by the matter can outrange the possible states in terrestrial physics by many orders of magnitude. This is on the one hand a difficulty if one aims to understand and interpret astrophysical events. But on the same time, it is an opportunity if one wants to test the universality and predictive power of theories developed from the observation of terrestrial phenomena. The wide range of conditions that are assumed in supernova models is illustrated in the two figures on the left and right hand sides. The color codes the number of times a (Density,Temperature)-pair has been assumed during a spherically symmetric supernova simulation that fails and leads to the formation of a black hole (left) or that explodes and ejects the outer layers (right). Two branches can be discerned: The one at lower temperatures describes typical conditions during core collapse, the upper corresponds to the conditions of shock-heated matter between the protoneutron star and the accretion shock.
The input physics enters the simulations in form of interaction with macroscopic fields (gravitational, magnetic), an equation of state for hydrodynamics and composition, a reaction network, or cross-sections for radiative transfer. We have contributed to the organization of the Workshop Data Needs for Nuclear Astrophysics, Jun. 23-25 2006, in Basel. Some tools to handle nuclear equations of state in hydrodynamical models are shared on our download page.